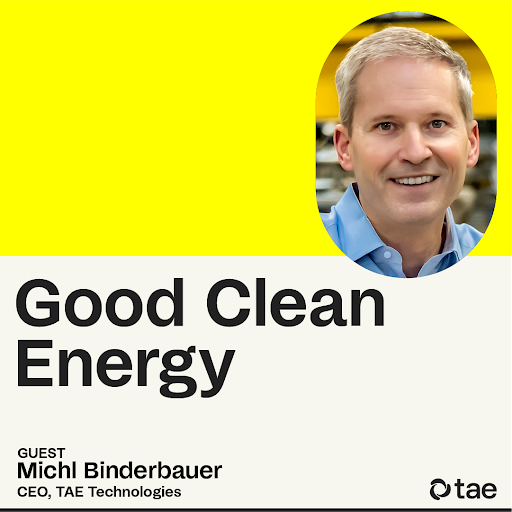
GOOD CLEAN ENERGY
TAE CEO Michl Binderbauer: The Path Ahead for Fusion Energy
Good Clean Energy is a new podcast about how to build a world with abundant, affordable, carbon-free electricity. TAE’s Chief Marketing Officer Jim McNiel hosts in-depth conversations with scientists, innovators and energy experts about the challenges our electricity systems face and the race for game-changing, clean ways to power our lives.
On this episode, Jim is joined by TAE’s CEO Dr. Michl Binderbauer to talk about the recent major nuclear fusion breakthrough and the steps forward to making commercial fusion power plants deployed around the world a reality.
Jim McNiel: Does this mean that your local power plant is going to be operating with fusion energy in the next year? No. Does it mean that we’ve proven that fusion can take place on Earth? Absolutely. I have with me today Dr. Michl Binderbauer, the CEO of TAE Technologies. He’s a physicist, a fusion expert, and he knows what it’s going to take to get to commercial fusion. We talk about what this event means and what stands between us and delivering a commercial fusion power plant…the pros and cons to the different approaches and how TAE is taking a road less traveled.
This is Good Clean Energy. A podcast where we discuss all of the steps that we have to go through to achieve a future of good, clean carbon-free energy. I’m your host, Jim McNiel.
Jim McNiel: Welcome, Michl. It’s good to have you here.
Michl Binderbauer: Thank you, Jim. Pleasure to be here.
Jim McNiel: This has been such an exciting week in the fusion industry, what happened at the National Ignition Facility on December 5th. Can you put that in context, kind of the history of fusion and what it means today?
Michl Binderbauer: This is basically a high point in this, what is it, 70 years that we’re chasing terrestrial fusion, something like that.
You know, this has been the aspirational goal. The first goal, frankly, it’s not the power plant yet, right? To sort of make more energy than what it consumes. It’s kind of the differentiator between what’s sort of theoretically plausible and most people in the field, of course, believe firmly so they dedicated their careers to it to sort of now have the proof in the pudding.
Right. It’s that definitive demonstration. Yes, it can be done terrestrially. It doesn’t just work in stars. You can actually do it here on the ground.
Jim McNiel: They are able to take what is essentially like a peppercorn size pellet of deuterium and tritium and excite it using x-rays generated by a laser, and I think it was 192 lasers in what a billionth of a second. And when those x-rays excited that pellet, what happened?
Michl Binderbauer: Yeah, that’s exactly right. It’s a massive installation. It’s football fields in size. If you ever get to visit it, I highly recommend it. It is a eye-opening thing of human ingenuity, you know, creativity, engineering, all this stuff. So yeah, so the lasers they basically all impinge on this super tiny target inside of what we call a hohlraum, which is like this cylindrical cavity.
That they shine into and that creates the x-rays that then impinge onto this, as you said, peppercorn size target. And then what happens there is basically the energy that deposits on the surface of the target vaporizes that material and that material explodes out. It moves outward from the surface. And by conservation of momentum, basically the rest of the material has to collapse and that leads to an implosion. And that implosion ultimately gets to a point where the density of this material compresses so much that it goes about to the density or beyond the density actually of lead or dense metals. And at that stage you reach higher temperatures and this very dense packing. At that point you reach the beginnings of fusion and then this early kernel of fusion occurring then heats the rest of the pallet. We call that ignition. That’s when the energy in one part of this tiny little amount of material is sufficient to then propagate that. And then the entire little pebble burns up basically and converts the deuterium and tritium into helium and also, of course, neutrons. And then those neutrons fly out and those are the signatures they measure. They measure the energy contained in that flux that comes out. And that was bigger in the end than the amount of laser light that they sent into the cavity.
Of course, we have to be careful here because we in the field understand this deeply. For layperson, it’s important to appreciate that this isn’t quite net energy in terms of if you look at a grid and how much energy went in the facility and then converted to the laser light and all that, we’re not at breakeven yet, but we call this scientific breakeven, and this has always been the academic first step.
You’ve got to get to that, which is the light, the energy, and the light that hits this plasma that’s formed, that that energy is smaller than the amount of energy that does eventually emerge from that little paper corn burning up.
Jim McNiel: So when you go down the commercial fusion path, you’re going to be held to a very different standard, which is a commercial Q, which is all the energy that you consume to operate your reactor versus the energy that that fusion reaction produces, right?
Michl Binderbauer: Yeah. It’s actually even more tight than that. Yes, it is correct. It’s certainly that, but it’s also, then remember the energy that comes out here is in the form of mostly the neutrons, right?
I mean, when tritium burns up with deuterium, you get copious amounts of neutrons. That’s where most of the energy goes, and the rest goes into these fast moving helium particles, but that’s the less amount. So now you have to do something useful with that. You have to get it into electrical energy, right? And so you have a second step, if you will, the envisioned solution there is that somewhere this energy that is in the form of fast moving particles, we call this kinetic energy in physics, converts to thermal energy and that thermal energy then gets converted into electrical and that goes through something we’re all probably somewhere familiar with, right? The idea that we make steam and the steam eventually drives a turbine and the turbine drives the generator, and the generator cranks, then come electrons. So there’s inefficiency in that as well, right? So if you’re looking at the overall of what’s needed, yes, you’ve got to include in your accounting all the energy from the grid throughout the facility, then the production coming out of the burning plasma core, and then converting that mostly kinetic energy into electric energy eventually.
And then hopefully there you have more than what you initially fed from the grid and that extra will go out to the world.
Jim McNiel: So Michael, this was inertial confinement fusion. And so this is a pulse of laser into a target, which is very different than magnetic confinement, which is a steady-state process. You want to walk us through what the differences are between those two approaches?
Michl Binderbauer: Yeah, absolutely. So you know, what they did at the National Ignition Facility was essentially a little microbomb going off, right? If you were to try to turn that into a power plant, what you would need to do is you would have to have, you know, a succession of those microbombs at the right rate to get an average energy out of the system.
And in the case of the National Ignition Facility, or at least in the design work of that, they’re thinking maybe they would need to fire this 10 times a second. There’s other sort of intermediate pulse systems they think they may have to fire once a second, but it’s a high repetition rate. Now what we are doing, on the other hand, is in steady-state magnetic confinement fusion, and as the name implies, steady state, there isn’t any one time event and then a break. It continuously produces, right?
So you essentially are heating up a plasma core and then a plasma core begins to fuse. So in the core, fusion starts to occur. And then you get energy out and you’re continuously fusing and you’re feeding new particles in while some are burning up. And so there’s a continuous loop that provides energy without any intermittency. In things like the National Ignition Facility pulse concept, there’s a break, right? It’s more like maybe the engine in a car, where each cylinder fires into a certain interval and then you get an average energy out to drive the car. This is the idea in these National Ignition Facility-like machines, whereas in TAE’s case, we’re looking to really run steady state.
Jim McNiel: Well, if you think about it, a magnetic confinement in steady state plasma is a lot more like the sun, except for the sun has the gravity and all its mass to hold that big ball of gas together. We have a cage of a magnetic field, which surrounds the plasma. And so it’s just that ball of plasma that we keep feeding and spinning and rotating, and that’s when all the fusion takes place right in the inside of that cloud.
Michl Binderbauer: Yeah, that’s exactly right. It’s actually a great analogy. I had never thought of it like this. I like that, the idea that the sun is, yeah, it doesn’t flicker, right? I mean, luckily for us, it’s constantly on, it constantly burns and burns and burns, and of course there’s so much mass there that, you know, it’ll take billions of years before it burns out and that same amount of mass is what holds it together, right? That’s how you get the gravity you alluded to.
And of course, that makes the whole thing work quite differently. You know, in the sun, the temperatures are actually lower than what we need in terrestrial fusion. For benchmarks, the core of the sun is about 15 million degrees. Terrestrially, we’re looking at things in the hundred million to a billion degrees, so it’s quite, quite hotter.
But the sun is much denser packed. The particles get compressed enormously by this humongous gravitational field. And of course terrestrially, we can’t do that. And as you said, that’s where we have to find other tricks. We use lasers like they did at the National Ignition Facility. In the case of TAE, we use magnetic fields.
And we try to put some pressure on, but it’s a bit gentler. And with that, you don’t get that same packing. And so we have to make up, because fusion is a product of both density and temperature. We have to make up with higher temperature therefore. And so that’s why terrestrial fusion is a bit different in terms of its regime, but it’s the same process. You know, taking the light elements, you bang them hard and you get heavier elements and you get a lot of energy in the process.
Jim McNiel: Considering the sun has about a million times the mass of the Earth, there’s no way we’re going to get the kind of gravitational force you get from the sun. So you have to make up for it with heat, right? And that’s why we have to get to temperatures of a hundred million to 150 million C for deuterium and tritium, and we have to get to a billion degrees for boron. So let’s talk about that. Let’s talk about neutronic fusion versus aneutronic. This was a DT [deuterium tritium] event, correct?
Michl Binderbauer: Yes, this was, and of course, you know, if you look, as you just said, the stepping stone, the ladder to the higher temperatures, DT is sort of your first step on the ladder. That’s when you get to leave your baby shoes behind and you’ve taken your first step.
So that’s at a hundred million, 150 million degree mark. That’s where deuterium tritium burns, and that’s what the NIF or the National Ignition Facility has done. That’s what pretty much everybody in fusion is aspiring to as the first step. And even in the case of TAE, well this isn’t our first step and we’ll talk about what we’re going to do in a second, but it’s also a step on the way. You have to get through that temperature regime.
And so, you know, you can put a checkbox there and then eventually, if you can do beyond then you get to the exciting part, which is what we call the aneutronic fuels. Now what does that mean? So those are fuels where there are no neutrons produced. Like I said a little while ago, deuterium tritium, as in the case of the National Ignition Facility, produces neutrons. And those who have been in the nuclear world or write about it will know that neutrons are sort of a bit of a nasty thing. They cause radioactivity, they cause radioactivation and radioactive decay. And so if you make neutrons, you have an impact on material. like little billiard balls or maybe little machine gun pellets hitting the surface of a reactor. And if you have enough impacts from these small bullets or projectiles, you eventually really destroy the integrity of the material.
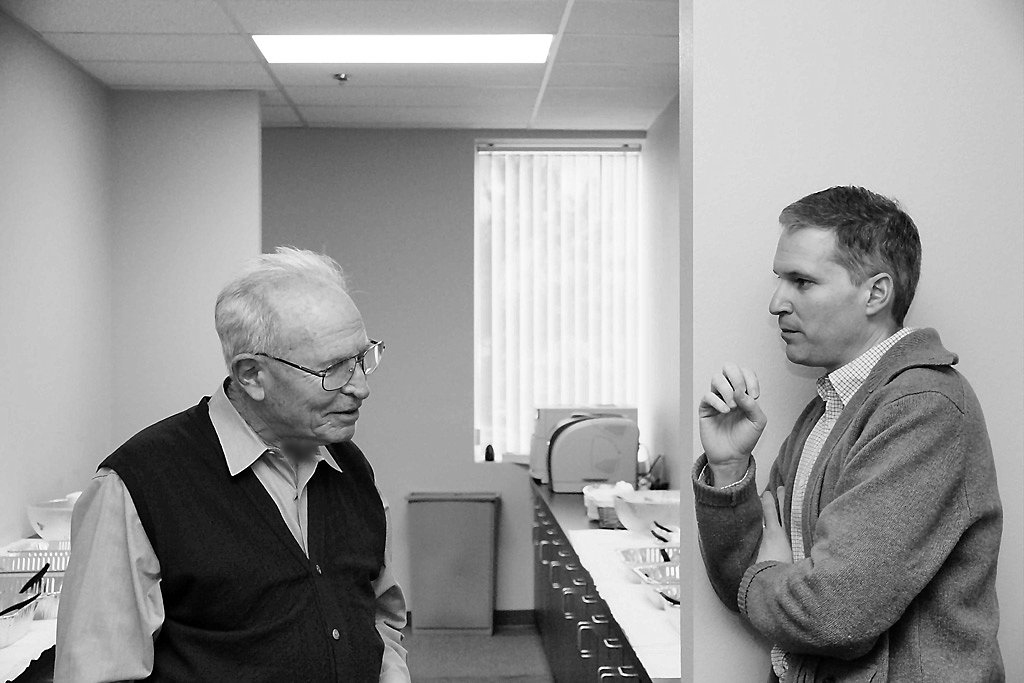
Jim McNiel: So Michael, from the very beginning, even back when you joined TAE, I think a mission of the company was to think about the end in mind, the end being achieving commercial fusion, a machine that is not just environmentally sound and intelligent, but also economically viable. And is that what’s really driven the mission to go down the aneutronic path and to use boron instead of deuterium tritium?
Michl Binderbauer: A hundred percent. I have to admit here, immediately that credit goes to Norman, right. When I joined as a student, completely naive, I mean I had no idea about any of these concepts frankly. I knew what fusion was to the degree that I had one course that actually I took from him as an undergraduate in plasma physics. But the notion was right there that one had to find a more practical and what that all implies meaning economic and you know, from a maintenance perspective and in every sense, something that fits the industrial setting of a utility. And fusion at least as was practiced in most laboratories in the world—government and to a degree private—certainly back then did not meet any of those criteria frankly. And one can’t really criticize for that. It was really the idea first, small steps for small feet, right? You got to get to net energy, like we just said. And Norman deserves a lot of credit because he went bolder and in a way demanded more of the program or more of the goal set. And when I started he said, Look, if we really want to make this an applied end product, meaning a power plant, then we gotta look beyond deuterium tritium. And that eventually led to becoming convinced that hydrogen and boron is something that’s doable which by the way, you know, in those days a lot of people, and even today probably, doubt that that can be reached because you know it’s about a factor of 10 higher in energetics than where you need to go for tritium.
But we set that as the goal and it defined pretty much everything else from there. So you can certainly say TAE was started when we were working at the university was about 1990 when we began working on this, it was all borne out of the idea that we had to engineer and design and create something that meets up with the end in mind requirement, which is economic clean power from fusion and practical and all those other things.
Jim McNiel: Well, you know, Norman was quite the renegade when you think about it, right? I mean, because everyone in the industry has been kind of revolving around the Russian donut or the tokamak. And from the beginning of TAE, it’s always been the field reverse configuration model, which remains today. Although you’ve learned a lot in building reactors and testing and building plasma, but it’s essentially his vision from the beginning, isn’t it?
Michl Binderbauer: A hundred percent. I mean, I think the remarkable thing is when we unpack a little bit further back in his career, he worked on a lot of those standard things in the field. I always say this, and this is no joke, you can’t get a PhD in this field without suffering, you know, through a lot of stuff that he discovered. You have to grasp those concepts that he discovered and published and, you know, researched. And so he was as much a standard bearer and a founder, if you will, of the field. I would think there’s probably, I’ve never counted them, but probably see a small club of what had called the popes of the field, right?
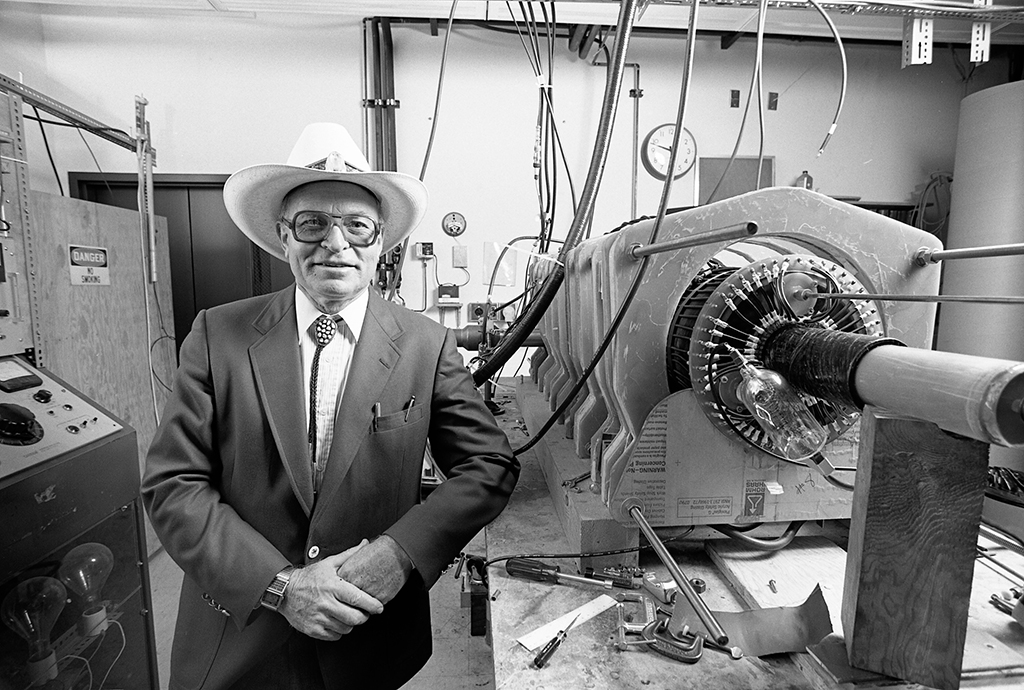
He did. And he took actually a lot of hits for that. I think everybody obviously revered him for what he did in his earlier part of his career. In his later years, some people questioned, said like, well, maybe he fell off the edge a little bit with this idea of, as you said, field reverse configurations and hydrogen boron and so on.
And so when I came in, it felt like these little sticks swimming against the current.
Jim McNiel: Well, you know, to be clear to people who are listening to this for the first time, a tokamak is like a hollow donut or a hula hoop, if you will, and a FRC or a field reverse configuration reactor is if you cut that donut in the middle and made it stretch out straight and pinch the end so now you just have a hollow cylinder, which has a vacuum. And then you fill that with a gas, and then you activate that gas by basically shooting what, hundreds of thousands of watts of electricity into it, right? And then it gets contained by this magnetic cage, which are the magnets around it. And then what happens?You’re, you’re gonna feed it and you’re gonna spin it, right?
Michl Binderbauer: it’s biggest difference actually is it makes most of its own magnetic field, right? So if we start there, in the world of magnetic confinement, most constructs and concepts people work on are defined by the geometry and the geometry of the magnets, right?
As you mentioned, the tokamaks, it’s a donut shaped device. It looks like a donut. There are multiple kinds of magnets that you need to affect that geometric topology and try to hold the fuel together. There’s a high degree of complexity, and the magnetic fields have to be very, very high, and they’re all applied from the outside.
Plasma makes some magnetic fields. You know, anytime you move electrical charges, you get a current, and the current, of course, generates a magnetic field, so you have that. But in the FRC, what’s remarkable is that, I mean, you need some outside magnetic field to sort of anchor this and give some level of pressurization, but a lot of it, and in fact, the predominant magnetic field actually gets generated by the currents in the plasma.
We call this self confined or a self-created magnetic field. It’s quite a different concept. And one of the handicaps, in fact, early on in that was that people thought it would be too unstable. You can appreciate if you’re relying on internal processes to kind of align themselves nicely and create the current that you want so that the magnetic field is there, how stable can that be? How homogeneous, you know, I mean, can you control all these parameters? Is it going to remain where you wanted in the amplitude you wanted? And frankly, to be honest, the early experiments didn’t look too good. You know, FRCs were very unruly. In a way they’re sort of little children, you know, they’re very energetic and they just break out of the cage and they hit the wall and the party is over.
A good friend of mine and another Norman student, and he’s our Chief Science Officer, Toshi Tajima, he always likes, he’s Japanese, he loves analogies. And he used to always say, FRCs they’re more like human beings where the rigidity that gives us the ability to walk and stuff is from our skeleton but that’s on the inside. If you look at the world of insects, for instance, you know, as he says, there’s the bugs, right? They have an exoskeleton, the skeleton on the outside and the soft stuff is on the inside. In a way you could argue then, you know, tokamaks is sort of like these insects and, you know, we are more like sort of a mammal and the consequences are, our soft stuff on the outside is pretty fragile, right? But it’s also very flexible. And as we grow, we don’t have to constantly shed our exoskeleton. And so there’s a lot of flexibility from it, but it brings internal dynamics. And the tokamaks in that sense they’re more constrained, they’re forced from the outside with the magnetic fields and we have to make sure we maintain the insides coherently.
And that took a lot of time. And now we, of course, are very stable. Those early problems are completely behind us. And Norman is not only vindicated but was absolutely right. Those things can be made very, very stable and very successfully get you up the ladder in performance towards the fusion regime.
Jim McNiel: Well, I guess it’s a bit like a spinning top right. The faster you spin it, the more stable it gets. And you once told me that the interior stack of magnets that’s inside of the ITER tokamak, that one of those magnets is strong enough to lift an aircraft carrier.
Michl Binderbauer: Yeah. Well that’s a good metric. I’ve never calculated to be honest, but that’s been in our community, sort of a comparative to say, yeah, it’s a serious magnet. It has serious forces that it has to sustain. It’s a wonder of engineering. And in fact, General Atomic is delivering these components to ITER right now. They’re building them down here in San Diego, about 50 miles south from where we are,TAE. And it is a massive engineering job, never before done in the scale that they did. And that’s a remarkable accomplishment, but it also speaks to the complexity and the high cost of these, right? You contrast that what we need, our magnets are more on the scale of an MRI machine or so, right?
A little bit bigger than an MRI because we want to make a plasma. It’s about a meter in diameter, maybe a meter and a half. Humans are a little smaller when we go into an MRI machine. So the bore isn’t that big, the tunnel in the middle, but the shape, the geometry, those are all very, very similar.
And the field strength is not that far off.
Whereas in the tokamak you need much, much higher field. And coming back to the other thing you mentioned about the spinning tops, that’s a great analogy and I always like to use that to sort of explain why one can make things that seem unruly, actually stable, right? We all play with tops when we’re children and we explore on how they spin, and they’re very nicely standing there, top heavy, you know, in the gravitational field doing their spin, and then they start slowing down because the friction with the table starts to sort of break them a little bit and eventually you start to wobble and then they flip over, right?
The electromagnetic analog is frankly an FRC and it wants to do that. The thing that prevents it from doing that is what you mentioned, it’s rotation, right? Another way to say it’s gyroscopic effects. You play with a gyroscope and you remember you want to turn that a little bit. There’s quite a bit of force that resists you and we call this conservation of angular momentum that builds that sort of counter torque there.
We use those kinds of principles actually exactly in the FRC, and that’s what eventually gives its stability and rigidity and the predictability that we need in repeatability so we can operate with it on a continuous basis.
Jim McNiel: Well, anyone who ever remembers learning how to ride a bicycle understands that it’s much easier to ride a bike when you’re going at speed than when you’re going slowly. Right? It’s a much more stable device. So we have less expensive magnetics because we let the plasma do a lot of the work in terms of creating a magnetic field as it gets more excited and gets hotter. So our cost for magnetics goes down.
But then there’s this whole difference between boron and deuterium and tritium, which is you don’t have to deal with neutrons when you have a boron hydrogen event. Certainly not at the level that a DT machine does. That’s a big economic savings as well, right?
Michl Binderbauer: Yeah, that’s exactly right. The economic savings come essentially from two things. As you just said, the magnets that are lesser and magnets are always a big expense in these machines, you know, half the cost of the equipment perhaps. It’s a big impact point. So if you can make the magnets less, then you have a big savings there. And then we have, we didn’t talk about this much, but because of the weight of the topologies and that most of the field is carried on the inside, plasma itself, there’s a higher degree of efficiency. And the efficiency of this is what also brings a big economic benefit. And then you go to boron, that is obviously extremely additive to good economics because now as, as we said earlier, it’s aneutronic, right? So you do not end up having to worry about what happens with neutrons. You don’t have a lot of equipment damage therefore. You know, plant life is not limited by this plasma thing. It’s limited by the normal things that plague anything humans build. And we estimate based on analysis of sort of engineering components, we will get the same plant life that you will get with the gas turbine or you know, any other generation technology of today.
So that’s economically a big difference than compared to say, a tritium based system where you will have to replace good chunks of the machine that are expensive and complex with robots, mind you, because it’s radioactive after some time on a continuous basis—continuous meaning like once a year or so. That only means you have to expend money and cost to replace parts, but the machine’s also down. It’s not going to make energy. So those things are big drivers. And lastly, the other economic driver is fuel itself, the availability. You know, if you look at tritium today, we have about 50 kilograms or so in the world. That’s not very much. You can almost fit that in a small suitcase, and that’s all there is. And that actually is coming out of fission reactors, believe it or not. And these are many of the ones that are conducive to making more tritium than others. The Canadians have a good fleet of those and those are at the end of life.
And so as those go away, a lot of the free tritium in the world will reduce. And it also decays if you don’t constantly replenish it. So there is a big impact from scarcity, even eventually on the level of experimentation. Not for now: There’s plenty of tritium here to do these experiments. You need very little. But eventually when you start reactors at scale, there is. And then you come to the cost point. What is the scarce material like tritium cost? Last estimate I’ve ever looked at were somewhere in the $30,000 per gram. This is super expensive stuff, you know?
Jim McNiel: The other thing we talked about once before, which you pointed out to me, which is really interesting, is you don’t need railroad rails or highways or pipelines to feed a boron plant. I mean, you could deliver the fuel for a year in the back of a pickup truck.
Michl Binderbauer: Yeah. I mean, you know, in a way that’s probably true for all fuels. There’s so many, you have them, and they’re available and the radioactivity doesn’t bother you and the classified nature of tritium, I mean, it’s also used in some of our thermonuclear warheads.
Boron doesn’t have any of that problem. First of all, metric ton mining: We live out here and our facilities out here in California. In the deserts of California, there’s actually a town called Boron, USA. It’s the middle of the Mojave Desert. They mine by the metric ton. Remember Borax 20 mule team, the little boxes of detergent, right? That’s exactly what we’re talking about. And so this substance is in ubiquitous supply worldwide. There are very large deposits pretty much everywhere. There’s no one concentration of it. So it’s really nice from an energy security or availability perspective.
And then when you look at, now back to your point about the power plants, it doesn’t consume much. This is the other beautiful thing that’s true for all of fusion, but particularly here, you need very little. When you take one fusion event, say you’re fusing deuterium with tritium, or you’re fusing hydrogen with boron. It’s nearly a million to up to 10 million times more energy release than when you burn a molecule of gasoline. It doesn’t take much to make a lot of energy is the point. And so when you look at the fact that ours is clean and nonradioactive and so on, you could definitely throw it on the back of a pickup truck the fuel you need for a year’s worth of operation of a machine that would make something like 300, 400 megawatts of power, which is, you know, what you need for a smaller city or town.
So, absolutely. And this, when you sort of scale that into where we need to go, particularly the developing world, where most of the demand is going to come from, that’s fantastic. As you said, you don’t need rail lines, you don’t need pipelines. Maybe you use a helicopter and if you can’t, you could hand carry it in. Joking here.
Jim McNiel: So let’s talk about some of the pitfalls of the fission industry. You know, we had our first fission plant operate in 1951 in Idaho, and it wasn’t until the late seventies when we had a hundred gigawatts of power online. So it’s not exactly what I’d call a rapid adoption of modern energy technology. But the point being is that every fission plant that’s built out there is built bespoke. It’s built as a custom thing. And there’s a lot of money that goes into shielding because obviously uranium and plutonium have half lives of thousands of years in terms of radioactive waste. And there’s also waste pools at these plants. We’re not going to be approaching it this way.
I think it would be good for you to explain to people the difference between — radiation’s misunderstood, I mean, obviously uranium and plutonium are highly radioactive. Tritium, much less so. It’s going to have a much shorter life than uranium and plutonium. And then even though we’re using boron and hydrogen, there is still a level of radiation. But as you explained to me once before, it’s kind of like ambient radiation. Can you talk about that for a minute?
Michl Binderbauer: Yeah. I think that’s a very important subject actually. I think hopefully this is something people can take away. Fusion and fission are sort of cousins, if you will. I mean, very odd cousins perhaps, but in the taxonomy of things, they’re both nuclear energy. But they’re very, very different.
So in the case of fission, of course, as you just said, uranium and plutonium, heavy elements are part of that game. And that means you have not just radioactivity while it runs, but you have a lot of waste material that has, we call this in physics half life, right? So it kind of describes the decay constant, which gives you a sense for the timescale over which something is radioactive. And in those heavy things, some of them in particular, you have timescales that you measure on the order of 10,000 years.
So you know, I’ve said this once before and I know people don’t like that, but I stick by it because I think it’s true, it’s a little bit of a pact with the devil, right? I mean, once you sign up and you get this stuff, what are you going to do with it? You’re a bit stuck with it. And 10,000 years, you know, I used to say this to people: What are the oldest things we have ever built that we still have quality construction? Maybe the Pyramids or something. You’re looking like, what, 5,000 years or so? And so now you’re up for something that’s supposed to be there for 10,000 or more. And it’s not just the structure. You have to cool this stuff inside because as it radioactively decays, it generates heat, right? So you got to cool it, you got to monitor it. You don’t want people to get to it. Can we have a government stable enough for 10,000 years to monitor the site, not forgiving the engineering and all that. Those are all big headaches and this is why of course the fission industry faces lots of problems. And this is just on the waste stream right now.
Contrast that to the waste stream on fusion. And it’s remarkable because what we find there is, it’s a bit radioactive if it’s tritium. If it’s hydrogen boron, it’s not radioactive while it runs. When it stops, worst case you will have stuff that have short half-lives. You know, in our case, for instance, in hydrogen boron, it’s never a hundred percent clean, but it’s almost clean.
And the few things that you may have, they have like half life, like 50 days. And it’s not radioactive to begin with. It’s more like a medical isotope facility. You know, the kind of things we find if you go into nuclear medicine department of a hospital today and you do a PET scan if you’re an oncology patient or something, hopefully not. But if you were, then you have to get these scans done and they inject you with small amounts of radioactive medicine. And these are in every hospital and they generate sort of a small waste stream. And that’s about the waste stream that we would find coming out of one of our plants. These hospitals sit in densely populated city centers. Nobody worries about it because we don’t have to. The safety there is not in question. So that’s a wonderful attribute of all of fusion. And when you come to hydrogen boron fusion, it’s just incredible.
And the other part is when you now think about what could go wrong while it runs well, then we think immediately when we think nuclear, right? We think Chernobyl. We think more recently, Fukushima. Those horrific accidents were within. You know, the core melts down in the fission plant and you get all this material come out, and then you have to quarantine the area for decades and maybe longer, and it turns into a wasteland, so to speak.
Fusion doesn’t suffer that problem. It’s really important people appreciate that. There is no meltdown possibility. I always like to say, what makes it so darn hard to do makes it incredibly safe. You know what’s at stake here, right? So we have to hold this super hot material together for long periods of time, and if anything goes wrong and the hot stuff makes contact with its surroundings, it cools off.
Why does that happen? Because while we make things very hot, we heat up very few particles and around it is all this dense cool material like metals and so on that are room temperature, right? So if those few hot things come in contact with many cold things, the heat transfers readily, and in fact, the cool stuff doesn’t really get any warmer. But the hot stuff cools out and gets cool instantaneously, so it stops. So the point is, fusion is a driven process. You have to constantly put energy in, you have to constantly massage it to keep it going. Fission, on the other hand, is a chain reaction process that once you start it, it self propagates and it doesn’t stop until everything is consumed.
And in particular, if it starts to run away on you, then you get a meltdown. And in fusion, everything stops instantaneously. Now I wish it wouldn’t be quite as severe because then we would have fusion already because that’s super hard. That’s where the challenges are. It’s hard to do. But once you have it going, keeping it going is even harder. And so if anything goes wrong, it stops. And so there’s inherent safety built in by nature.
Jim McNiel: What comes with that different radioactive profile though, is really worth considering because you can see a future where you can build proton boron reactors in a factory and ship them around the world. And have them stood up and commissioned and operational in virtually any part of the world, like in the dense center of Delhi or in Singapore, because you don’t have the same radioactive shielding considerations you’d have for a fission plant or even for a deuterium tritium plant. So from a deployment standpoint, it could be really commoditized, I think, much more efficiently than fission ever hoped to be.
Michl Binderbauer: That was exactly one of the key reasons we pivoted to hydrogen boron in the entire concept. It’s precisely for those reasons. You can make something that is very practical change the way power gets made in the sense that you do it where the population center or where the consumption center is. It could be for industrial use, could be for residential use, whichever it is. But you put it where the end users are, where the load is. And if you look at it today, I mean fission is one example where the plants are somewhere where of course nobody lives in case something goes wrong. But even look at renewables. I mean they don’t typically, I mean with a little bit on our rooftops, but that doesn’t sustain a city like New York or LA. You need much more power than that. And then you have to go out into the deserts of California, for instance, where you’re in the Great Plains and those areas are very sparsely populated. And then you have to bring that power to the, for instance in the US, to the coastlines, right?
So now you’re facing long transmission lines. Those are not only expensive and they are kind of ugly and they, you know, impact the environment, but they also are lossy. You lose a lot of the power. Now, contrast that with something like fusion. That’s high power density, very clean, no radioactivity to speak of if it’s hydrogen boron. You can place those in the consumption center. You get rid of the long transmission lens. What you really end up having is a much more autonomous grid, decentralized, many smaller plants perhaps. And you don’t have transmission in the utility anymore, really. You have mostly in the distribution, which, you know, they, that refers to sort of the last lake of things from, normally you have transmission lines from the power plant to some distribution side that’s near the city center or in the urban vicinity.
And from there they distribute it to all the little things like our homes and the industrial sites and so on. But with a more centralized plant, you don’t need that. So you get a lot of better stability, you get more resilience in case something goes wrong and you have more options basically.
And so reliability in the end goes up and the costs are lower and you will have less losses. It’s more efficient. I think this is absolutely a future. And if you look at urbanization and the trends we have where more and more people live in evermore dense centers, you will need sources of power like that.
It’s very hard to envision the mega cities of the future being run by low energy density fuel that has to be sort of shipped in, quote unquote, through long transmission lines from somewhere else. When you’re thinking of reliability and us as end consumers, what do we expect? Every time we flip the switch, the light comes on, right? If they ever send you a note on your email, on your text messaging and it says, Hey, we may have to take your circuit out, even for maintenance or something, it happens once a year, you get very upset.
Energy is so existential, electrical energy, to our quality of life, survival. If the fridge goes out for too long, things just goes south, right? These are all things that are sometimes maybe annoying, but sometimes really existential. So, reliability is a huge deal. And again, this isn’t trying to displace renewables or argue that they don’t have a space to be part of it. They do. They’re fantastic in that they’re green, they’re free of carbon emissions and they’re getting cheap. So they’re from a cost point attractive, but they do have that one problem, right? Mean they have two problems really. They have the low energy density for one, and the other one is of course variability. And we’re a bit at the mercy of mother nature, of the weather. You get it when the sun shines and when the wind blows and you don’t get it when those things are not on. And we now know, and we have enough weather data, for instance, in the US and I have a friend who studies this at UC Irvine, where they can look at any county anywhere in the country and you know, pull up the weather data for the last like say 50, 60 years. And you will find these periods where there’s, I think Boston or something, during those 50 years, there’s a period of three weeks without any sunshine. Dense cloud cover, what are you going to do? You have solar there, right? It’s not gonna not going to help you. Can you build batteries that can store all that? That’s one way. That’s very expensive, of course, and we don’t even have the capability today to quite do that, right?
The other solution, of course, is you have backup power. I like to call it sort of shadow capacity. It’s capacity that sits there that idles or it doesn’t even run, costs you a ton to maintain for those few moments when you need it, right? That’s economically not viable either. This is where fusion slots in, becomes that reliable available baseload power.
The utility sector calls this dispatchable. This is power you can always turn on and off when the humans want it. We control that, not nature, not the weather. It’s clear now in all circles that we’re going to need that. And if you look what’s available today to service that part of the electric supply chain, well it’s either carbon based things like, you know, gas turbines and so on, which we want to get rid of, or it’s nuclear fission. Today it’s the only carbon-free one that can do this baseload. And this is where fusion comes in. Once we have it, I think it and renewables will be the future of the grid at some point.
Jim McNiel: You know, it’ll be interesting when we have the final bill of materials in the cost of goods for a commercial nuclear power plant to compare what the material costs are for a 500 megawatt plant versus 500 megawatts of wind. Because a wind turbine takes about a ton of rare earth metals. And I think that when we get to that commercial level, we’re going to find out that fusion is pretty darn cheap to operate.
Michl Binderbauer: Yeah, I’m sure. I mean is, you know, this is of course always gonna be the battle on the statistics. Okay, are you talking about the first 10 plants or are you talking about plant number 1,000?
There’s a lot of things that will impact that, but you’re absolutely right. First of all, rare earth materials and those kinds of scarce resources out of the ground that we need to build some of these infrastructures for motors and turbines and so forth, that’s a big impact point. The scarcity of that will impact price negatively, right? It drives it up. It also leads to potentially more conflict. You know, it’s not just energy in itself, but the tools to make the energy that can lead the conflict. So, you know, you think of some of these rare earths or in batteries, what you need from lithium, the cobalt and so on. All those things are impact points.
And then of course you have the economies of scale where you build these things and deploy these things. And if you have systems that are more practical, that are more compact, that can be built with less complexity, that can be shipped at lower cost to sites, those are all going be there to make a big, big impact.
And then when you look at our opportunity with our fusion designs, these can be centrally manufactured. This is the vision, right? You have centralized facilities that will, at industrial scale, build these things, cookie cutter if you will, and then you ship them out to sites do final assembly there, of course, and installation. But this is very different. For instance, talking about fission and you said bespoke, it’s a great way of saying that, right? Because these plants are all, in a way, monolithic one-up designs that don’t find replication typically. The people that built them, the crews that do it, a lot of it is concrete and rebar that’s done site specific once with local tradespeople that do that and they don’t move on and build the next one and the next one, the next one so they learn and they get more efficient at it. That just doesn’t happen. When you centralize stuff, we know all the things we make cookie cutter, they do get cheaper. And so there’s no question in my mind that there is a point of inflection where fusion has the potential not just to be clean and in control of, in terms of from a reliability perspective, but can also really be in the most cost competitive spot on the generation assets of the future. Not plant 1, but sufficient plants built and learning curves down. and we’ll get to that.
Jim McNiel: So going back to our original opening topic, which is what happened at the National Ignition Facility in California, this is a major breakthrough event because it proves that you can get net energy out of a fusion event. Where do we go from here?
Michl Binderbauer: So we’re about to build generation six. This is a machine we call Copernicus. We hope to have that on and up and running somewhere around mid decade, say 2025. So ‘25, let’s say it starts to operate. Then we should very quickly get to a point where we can show, just like the NIF did, that the conditions are right to make net energy. And then the journey from there is pretty well defined. We have to build then one more upgrade to get to a power plant. Copernicus is still an experiment. We measure power in. We measure power out. We do some synthetic computer modeling on the what if, if we had all the conversion systems and we use the right fuel in it, and then what do you get and so on.
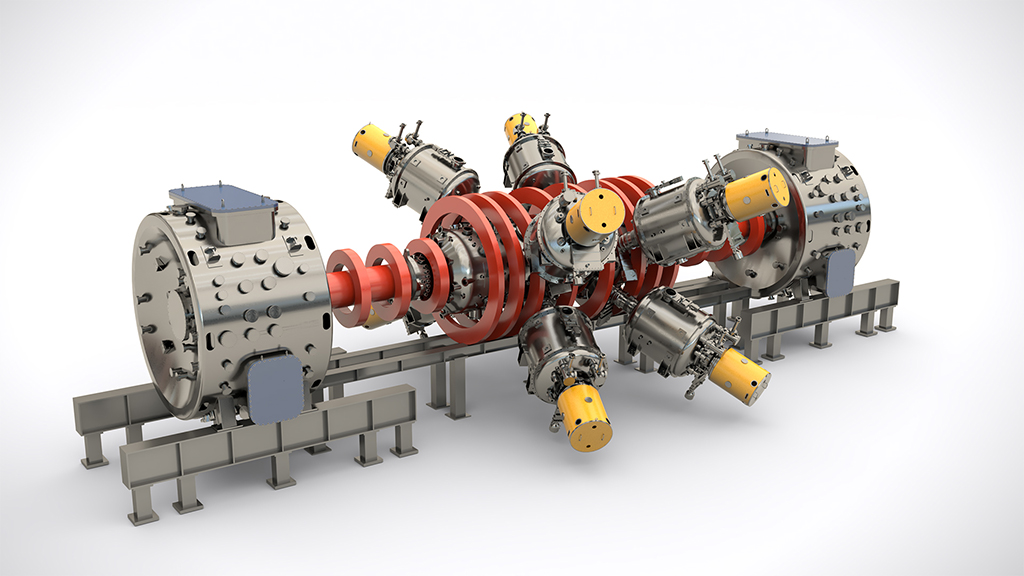
And that’s sort of then the dawn of going to the first steps of commercializing. So I think by the early 2030s, our technology should be in a position to build out the first plants that then can connect and will connect to the grid.
Jim McNiel: Well, it’s an exciting path and it must be really satisfying for you. I mean, you’ve dedicated your entire career to fusion and to have this event take place where, how many conversations have you been in before where people have said, Well, this is not going to happen. You can’t make fusion happen on earth. You know, there’s just a whole bunch of naysayers and doubters out there. To a large degree, this may put some of that conversation to rest.
Michl Binderbauer: It does. You know, I think it was, you and I had talked the other day about Roger Banister and breaking the four minute mile. Before that people thought, gee, can human anatomy lend itself to running at that pace? Maybe not. Right. Well, that doubt gets taken out now. And I think the naysayers or the doubters now have to take a step back and say, okay, you know, net energy terrestrially is possible and that’s a huge boost, not just because it’s probably going to bring more capital to the field, there’s a higher excitement and degree of confidence that you can take this to the commercial plants that will help convince those that may have stayed on the sidelines and said we’re not gonna invest at this right now, it’s too risky or too uncertain. There’s gonna be a change in attitude on that, but it’s also the emotional side, right?
Once you have an existential proof of something, it changes emotionally how we interact with the challenge or whatever. I’m not superhuman. I mean, there days were, I would sort of listen and my wife would say, Michl, you’re working so hard. Are you chasing ghosts? You’re at it for 25 years. I would say, no, no, no, no, I’m not. But the reality is, of course, somewhere you think about it. Gee, maybe this is a problem that’s too hard for humanity. That’s nice to have been removed. We now know it can be done. And I think as humans, when you look at successes of things like the moon race and us getting up, you know, in the late sixties on the moon and safely back, these are the kind of events where once they’ve happened, it’s an inflection point and there’s a whole different layer of confidence and conviction.
And it usually leads to more efficiency and better focus. And the emotional boost you get just makes you work faster, better, quicker, more accurate. I don’t know. It’s all those things, right? And I think you will see that now. There’s no question in my mind, whether it’s funding or it’s progress.
And just like in the case of the four minute mile, you know, after Roger beat the time, very quickly, I think it was like a couple weeks later, suddenly another runner did, and then another runner did. And I’m not saying in a few weeks we’re going to do that, but over the next few years you’re going to see that.
And I think that’s probably the most enduring thing to take away out of that event. That while maybe this particular kind is hard to convert quickly to a power plant, there are others of us that have a way to do that more appropriately. And we are now going to be empowered by that event. More funding will come. The government will be, I think, a lot more conducive to supporting it at higher rates.
I’m sure the people in Congress have paid attention to that and. As a nation, I think we’re excited now. Those things are no longer now the question. The question is now how can we get there fast and, you know, contribute to what we need to do, which is, you know, get ourselves off fossil fuels and create this world of abundance that is not conflicted with strife in the world over energy resources, but you know, how we bring them to everybody on the planet and improve qualities of life and access to things and so forth.
Jim McNiel: We need dreamers, we need explorers, but we also need builders and we also need followers. You know, we need them all. And when we think about the scale of the issue that we’re trying to deal with in terms of providing good, clean, abundant energy for everyone on the planet, and the fact that the demand for electricity is going to double in the next 25, 30 years, we’re going to need everybody.
If you made it happen tomorrow, Michl, if we discovered something and in next year, we had net energy out. TAE couldn’t meet the needs of this planet in terms of delivering fusion energy to every place that needs it. This is going to take an army.
Michl Binderbauer: Oh God, yeah.
Jim McNiel: Or not an army. It’s going to take a movement. It’s going to take all hands on deck.
Michl Binderbauer: It’s like they say, It takes a lot more than a village. It takes the planet. It’s a global thing, right? You know, people always ask me, Now that the National Ignition Facility did this, does that bother you? The answer is no, it doesn’t. I mean, first as a human, I want this solution.
You know, I’ve been now spending my career on delivering and developing that, or trying to do that anyway. And then either you think the field is small, the challenge is massive. It’s like, you know, we’re all little Davids going against this insane Goliath of nature, this challenge. So everybody that contributes something that gets us a step closer is not just a hero, but a role model in somebody you aspire to and cheer on.
And if more than one of us succeeds, to your point of the skill of what’s coming at us, we need all of it. I mean, think about what’s at stake. So I always look at this from sort of a historical perspective in the statistics of the data. We need to about double our electric generation capacity on the planet over the next 20 years or so. So by mid-century roughly, we need to be at double the available power production capability globally. Now, where we are today is already incredible, but think how long that took. That goes all the way back to the first steam engines in the Industrial Revolution. That’s taken us, what is a fair number, I don’t know, 150 years or so. You can pick a different definition where you start counting, but it’s a long time. Now we want to replicate that and compress that into like two decades. And by the way, not using combustible materials. We want to do it clean and in a very environmentally benign way. The burden is enormous and the scale is enormous.
Jim McNiel: It is enormous. But the thing that gives me hope, you know, because I was musing on the fact that 75 years ago this week, and I think it’s actually tomorrow, was the first successful test of the transistor. Was it Shockley? I think. Shockley, right?
Michl Binderbauer: Good point. Yep. Absolutely. It’s Bell Labs.
Jim McNiel: And then, you know, part of his cohort was Moore, you know, that created Intel and, you know, this was the birth of Silicon Valley and look where we are today. I mean, it’s been 75 years of taking transistors and miniaturizing them and putting millions and millions and millions of into this microphone, or into your phone or this computer. And part of me gets a little discouraged and I think, wow, that took a really long time to get to where we are today. But then I remind myself that what we have available to us just in the past 10 years is radically different than what we had when we started TAE. We have high computational computing. I mean, really super powerful computing, much more than we had even 10 years ago. We’ve got additive manufacturing, so we can 3D print and test and iterate on components within the walls of TAE in weeks as opposed to months. You have machine learning and artificial intelligence, which has definitely moved the needle a great deal.
Michl Binderbauer: The defining moment in why fusion now and the always standing joke around the field, right? It’s decades before and it’ll always be decades. Why it’s changing is really, it’s multiple things. One is, of course, we’ve gotten smarter about how that works and we understand it better. And our first contributors back in Norman’s generation and before, I mean they’ve laid that foundation. These are giants on those shoulders we stand on. But now when you look what we have, what these guys didn’t have, we’ve got tools that can deliver pressuring stuff with magnets, using intense lasers, using particle accelerators that didn’t exist.
You mentioned all these other technologies from machine learning to the fabrication techniques and stuff. All these things are now here. Some developed, like we did some of these innovations in our own history. Some came by serendipity and parallel evolutions elsewhere. But the important part is they’re here now and there’s a confluence of technology and tools with knowledge and wisdom, and it’s very exciting that that’s happening right now.
And so I think in the next five to 10 years, I think we’ll see some pretty explosive movement forward in a fast pace in the direction to get to these plants. I think we’re in an accelerated stage of delivery and I think everybody senses that that’s around the field. I mean, the people like us that work in it.
I mean, if I look back on my early career and you know where we are now, it’s not just the receptivity of our idea by others. The entire field is lifted up. You know, and it wasn’t just the event on the National Ignition Facility in the last couple of weeks, but there were other precursors like that. We announced last year some of the work we’ve done in machine learning with Google, which completely resets the timescale on which you can actively control plasma and manipulate things. I think people in Switzerland working together with the British outfit on machine learning did similar things in tokamaks now. Now there’s a capability now on feedback and adjustments that can happen on less than the blink of an eye, right? Those things are there.
You had last year, JET, the European Torus, show that they could hold plasma at very high performance levels together for quite a good long time and make megawatts of power in the process. And you had one of our private competitors—compatriots, I should rather say—Commonwealth Fusion, they showed these higher temperature superconducting magnets that could make a big impact on compactifying fusion machines. So there’s all that and in additive manufacturing, all this stuff. So yeah, it’s an incredibly exciting time and if I think through my entire career, had we had that 20 years ago, we would probably be further and but it’s good that it happens now and I’m still young enough and energetic enough to go flog at it for another decade plus. So another decade, at that point, hopefully I have a power plant.
Jim McNiel: You can cut the ribbon on a commercial power plant.
Michl Binderbauer: There you go.
Jim McNiel: Hey Michl, one last question: Have you talked to your kids about what happened at the ignition facility and what it means to your life work?
Michl Binderbauer: We did, we did. I mean, in fact, my wife is a big nerd on these things. She videotaped those TV interviews and stuff, and then, you know, kids came home from school and she said, Look, Dad’s on TV. And she said, Do you know why he’s on TV? And then they start asking, right? And so we start talking about it. And my son, he’s 12, and he’s very, very precocious. We had him and his class out a few years ago. We brought the entire fourth grade up to our facility and we threw a day of physics experiments and stuff. And when they got back, the echo from the teachers and the people of the school was, this was the coolest field trip that they took in their entire years, you know, five years in elementary school there.
And the kids were really, really energized. That’s what shows you, you know. And so they naturally started getting interested. And today if people ask, you know, What’s your dad doing? He says that he’s trying to save the world in a way. It’s overdramatic, perhaps but the kernel is there.
So no, they do understand. They did ask, and I explained and I said, you know, this isn’t something we did at TAE. But this is in a way a bootstrapping event for us too. And then I said, then stepping away from what egotistically your dad does or whatever, but from a perspective of humanity, right? That is an awesome event that should all of us feel really cheery about and moves us forward.
And I think certainly the kids understand that and they were very excited. And you know, if you ask them today what some of the problems are, they’re also much more educated than we were when we were growing up. I mean, to be honest, I feel pressure from kids. My son has put me to task and said, Why is it taking so long? He’s asked me this for the past couple of years. How come you don’t have a power plant yet? I said, Honey, it’s superhard to do. You know, this isn’t easy. But we talk about that actually quite a bit. And I find that fascinating that they, that generation, first of all, I think they’re already much more aware of the impact on climate and other things. They learn it now in school. I mean, you know, it’s amazing. We go out, we go hiking and there’s a little piece of trash or something and my son picks it up and I say, you need to be a little careful cause you don’t know who held that and you know what’s on it potentially. But he says, well, no, no, no, no, we have to.
If we leave that we’re going to trash this place. We have to be stewards. It’s remarkable. This was years ago when he was probably seven, eight years old saying that. And so they will take us to task, I tell you. And I think one of the things that to me is important to make me feel good, it’s a stewardship thing, right? We’re all here to contribute. and hopefully make this place a better place. It doesn’t matter who you are and what your political beliefs are, right? This is impactful from whatever perspective you look at it. Yes, you’ll have obviously for those who care about the environment, the ability to make that a better place.
For those who care about energy availability, on a more egalitarian basis between the have and have-nots—today there’s huge gradients, right?—we can fix those. For those who are national security and sort of hawks on that side, they will find that this is a way to secure energy for each country independently in a way, and for those want peace, that will bring peace then, because then we’ll have less rivalry over the scarcity of these resources.
So I think there’s no question about it. And when you look at an individual level, it brings prosperity, it brings health. Healthcare is expensive, but also energy intense. And in many parts of the world, there’s enough energy to even have a foundation to build good healthcare on top of it, right? So energy impacts just every facet of our lives, more than anything I can think of. And then derivative of that, all the other benefits that come. So yeah, absolutely agree with you. It’s a huge impact economically, policy wise, the fundamental behaviors of why we go to conflict with people is going to get very changed and perhaps hopefully goes away.
Jim McNiel: Well, Michl, I for one, want to thank you for your tireless dedication to this mission and your commitment to building this vision for TAE and realizing fusion—and I’m going to say this with great emphasis—in our lifetime because I think that’s what’s going to happen. I’d like to thank you for your time on Good Clean Energy.
Michl Binderbauer: Thank you. I much appreciate being here chatting. This was a really, really fun conversation.
Subscribe to Good Clean Energy Apple Podcasts | Spotify