FAQ
Frequently asked questions.
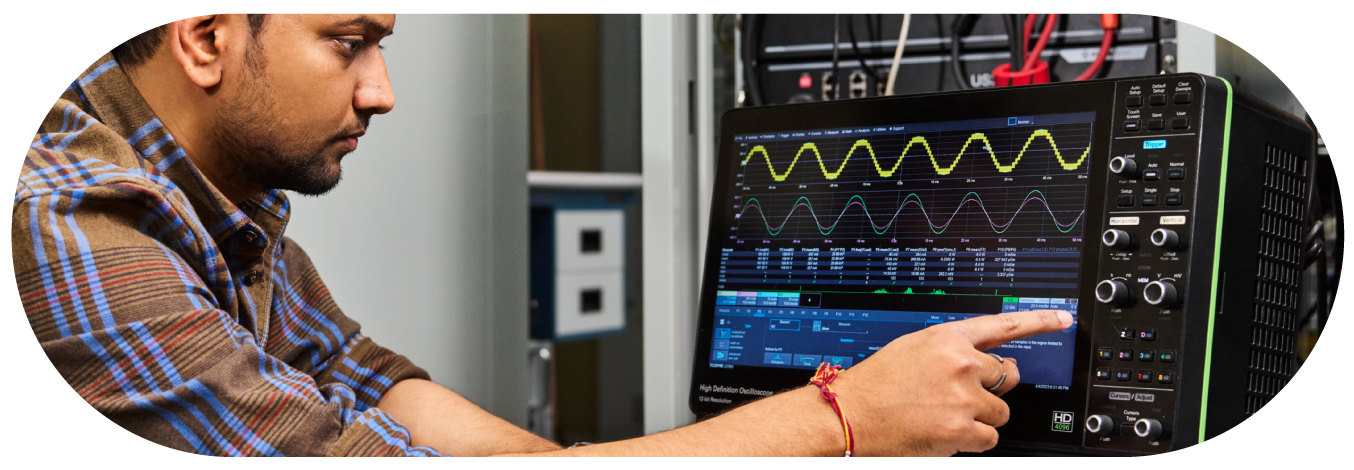
What is fusion energy?
How do you produce electricity from fusion?
Why don’t we have fusion power plants yet? What is the central challenge?
Generating power from a terrestrial fusion power plant depends on two conditions: maintaining plasma at sufficiently high temperatures and for a long enough amount of time to sustain the fusion reaction. TAE calls this the Hot Enough Long Enough (HE/LE) requirement.
For reactor performance levels, Hot Enough means reaching at least 100 million degrees Celsius, a temperature that is readily achievable today. However, sustaining that hot environment is extremely challenging because plasma is a delicate substance that must be protected from conditions that would otherwise decay it or cool it down. Terrestrially achievable plasma contains very few particles, especially compared to the particle density in the areas surrounding the plasma, such as the metallic walls of Norman’s containment vessel or even the air we breathe. Consequently, those areas can store much greater amounts of heat than what is contained in the plasma. These factors are responsible for the rapid loss of energy out of the plasma and into the environment. Fusion’s goal is to minimize this loss so plasma can be maintained with less energy than the fusion reaction generates. By doing so, net energy can then be released to the electric grid.
Over the past 50 years, the different fusion efforts around the world have worked to overcome this fundamental challenge in different ways. In December 2022, the Lawrence Livermore National Laboratory’s National Ignition Facility (NIF) recorded net energy gain for the first time in the history of fusion research, and repeated their findings again in August 2023. Read TAE’s statement on how this landmark achievement marks the dawn of the fusion age. Learn more about bringing fusion power to the grid on TAE’s podcast Good Clean Energy.
What is plasma?
What is TAE Technologies’ unique approach to confining plasma?
TAE Technologies’ unique approach to fusion combines the deepest insights from the two distinct fields of accelerator physics and plasma physics to tame plasma and solve the challenge of confinement. We use accelerators to inject beams of high energy particles into the plasma, which act as a “thickening” agent that makes it more manageable. As a result, this means fewer energy losses and more energy to release to the grid.
How does nuclear fusion differ from nuclear fission? Can a fusion plant experience a meltdown?
A fusion plant cannot experience a meltdown. Fission is propagated by a chain reaction. Once the reaction starts, it’s hard to stop. In contrast, fusion is a driven process, meaning all steps are deliberately initiated and actively maintained. Once the external drivers stop, the fusion process stops – faster than any kill switch or emergency power-off system could shut down a plant. Fusion possesses nature’s ultimate safety valve: there is simply no way for a meltdown to occur. In this way, fusion is an inherently safe proposition.
What is the difference between hot fusion and cold fusion?
The fundamental difference is in the parameter space of the fuel. In cold fusion, research is being pursued at room temperature, within tabletop experiments. The fuel used in cold fusion is approximately the density of liquids or solids. In contrast, hot fusion occurs in large, sophisticated machinery capable of achieving temperatures in the millions or billions degrees Celsius, and produces very low-density plasmas (100,000x less dense than air at sea level). Almost all of the world’s fusion research is focused on hot fusion.
What is a fuel cycle and how does it affect fusion?
How does p-B11 compare to other terrestrially achievable fuel cycles?
Benefits: The lowest temperature for a fusion reaction to occur; fastest reacting fuel cycle, with very large energy output per reaction.
Challenges: Tritium is a radioactive element – it does not occur in nature and must be bred; its associated neutrons will accelerate aging in power plant materials.
D-He-3
Benefits: Substantially less radioactivity and production of tritium than with D-T fusion, leading to longer power plant life; most energy output per reaction, largely in the form of energetic protons, which makes direct energy conversion possible.
Challenges: Residual radioactivity; reacts slower than the D-T fuel cycle; no terrestrial He-3 resources – must be mined on the lunar surface.
p-B11
Benefits: Aneutronic (primary reaction yields no neutrons); cleanest, safest, highly abundant, and environmentally friendly fusion pathway; enables scalable, cost-competitive electricity.
Challenges: Requires superior confinement and operational conditions to reach the considerably higher temperatures needed; reacts more slowly than other fuel cycles; less energy output per reaction.
It's often repeated that “fusion is always 15 (or 30 or 50) years away.” Is that true?
Why has there been a recent proliferation of private fusion companies?
With more awareness about the need for an environmentally benign, high power density energy solution, and with thought leaders such as the late Stephen Hawking strongly advocating for fusion as a permanent clean energy solution for the planet, it makes sense that more companies are joining the field. In recent years, new technologies have come into existence that provide foundational capabilities to accelerate development and fundamentally enable fusion power.
Advances in machine learning, artificial intelligence, computer-controlled power management and superconducting materials – to name just a few – all make the achievement of fusion inevitable. That potential leads to more investor interest and financing, which, by extension, leads to the awakening you’re seeing now.