TAE’s unique approach to fusion, with TAE CEO Michl Binderbauer
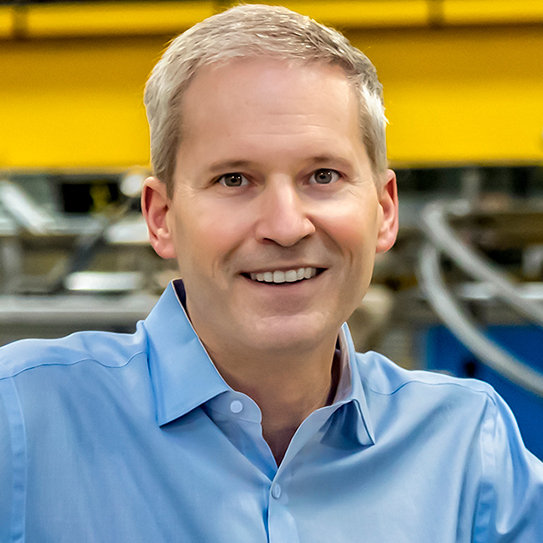
Listen and Follow ‘Good Clean Energy’
Good Clean Energy is a podcast that tackles one of the most existential questions of our time: how to build a world with abundant, affordable, carbon-free electricity. This season we’re going to unpack all the things that TAE is working on to make fusion energy a reality.
In this episode, TAE CEO Michl Binderbauer dives deep into a specific fusion machine design called the Field-Reversed Configuration that TAE has pursued since its inception. He explains how the FRC works, its unique advantages, and how it differs from other magnetic-confinement approaches by allowing the plasma to generate its own magnetic field, reducing the need for expensive and cumbersome external magnets. Binderbauer details the difficulties of working with FRCs and describes the breakthroughs TAE has made in simplifying and stabilizing the process, paving the way for more practical and economically viable fusion power plants.
Covered in this episode:
- Understanding the field-reversed configuration
- Challenges and solutions in FRCs
- TAE’s innovations to improve FRCs
The following transcript has been edited for clarity.
Jim McNiel: Welcome back to Good Clean Energy.
This season, we’re really going to dive deep into how fusion works and what we have to do to get to commercial fusion power plants. I’m your host, Jim McNiel. It’s been said many times before that fusion is the holy grail of energy. Well, what’s the holy grail of fusion? There’s a lot of different ways of doing it and one thing you have to think about is machine design.There’s one specific machine design called field-reversed configuration that achieves things that no other magnetic confinement approach can achieve.
Today, we’re going to talk to Michl Binderbauer, the CEO of TAE Technologies, who’s going to break down and explain what a field-reversed configuration (FRC) is and why it’s so different than everything else out there.
McNiel: So Michl, can you explain what an FRC is and why it’s so fundamentally different and why it’s always been TAE’s prime objective?
Binderbauer: Absolutely. Looking at why the FRC is important, it solves every ideal sort of metric you would want to solve for in terms of a power plant. When you think of a power plant, first of all, you think of something that robustly runs all the time, something that is easy to maintain. Something that has to be cost effective. It has to run at the current rates of expense that utilities can competitively introduce the technology in the market.
The FRC is a unique configuration in the magnetic confinement zoo. It’s one where you don’t use outside magnets to create the majority of the magnetic field. Let’s pause there for a moment. This is sort of an abstract concept, but what does that really mean? What that means is that you don’t have to deploy a lot of big magnets that are very expensive to make, that are cumbersome to schlep around in the logistic systems that are hard to deploy and maintain. The FRC is confined from within. The plasma makes its own magnetic field and that field cages it. In that sense, it’s ideal, right? Less gear, you need less material, less cumbersome manufacturing, less cost, less maintenance.
The thought then obviously is right away, why aren’t the people all doing it? And then the reason is it’s harder to do when the plasma makes its own currents and makes its own magnetic field and cages itself, so to speak. Just think what happens if the plasma does something slightly different than what we wanted to do. It’s own sort of dynamic beast. It’s like an unwieldy child, right? You tell the little kid don’t go over there, but they tempt and they go there. The plasma in an FRC kind of behaves a little bit like that. So what you have to learn is how to manipulate that through feedback control and coercing and cajoling into behaving the right way. And that’s been exceedingly hard.
And over the decades, a lot of people in the field who saw those merits I just described, and clearly wouldn’t argue with anybody that those would be ideal if you could make them work, have shied away in the end and said taming this, making this confined to the way we humans wanted to run indefinitely and in full control is exceedingly hard.
McNiel: Okay So the FRC has always been the goal. Where do we stand in terms of mastering the FRC?
Binderbauer: So the FRC has sort of two things that are challenging about it. One is it’s an odd kind of configuration, right? You have a magnetic field that you apply from the outside that…for those listening, magnetic fields have directionality and they have a polarity to it. So let’s say you’re in this cylindrical tube and you have a field that runs from the right to the left. And then that’s just a simple straight down the tube kind of pointing set of arrows. And if you now introduce current into this layer of this magnetic field that’s there, and typically what we do is we introduce this ring current that sort of sits inside that tube and it circulates the plasma, makes this current. Then that current creates its own magnetic field. So you’ve got this enveloping cocoon of magnetic field lines around that and that bubble of field pushes out the primordial field you do from the outside. And this creates this FRC topology? So one of the problems is how to make that. When you begin with just this primordial field, how do you get all this thing to happen? And historically people had only one solution, which was sort of like in a way use a big sledgehammer.
And what that meant converted here now into this field is you made two smaller kind of FRC-like objects. You can accelerate these things. You keep squeezing at the very ends of the field and you accelerate these two blobs of plasma with their magnetic field embedded. And then they were supersonically colliding eventually because it got accelerated sufficiently. And so it’s a violent collision. The collision creates a compound object and that becomes the FRC.
And the disadvantage with this is because it’s a bit brute force, you cannot quite control the intermediate process. In fact, I would argue it’s so short, it’s so quick, you can’t control it really at all. But you kind of know where you start and you roughly, or to a good degree, know where you end, but you can’t really fine tune it and there’s a lack of control capability there and it’s inelegant and you have to have these starter systems and make that and they collide.
And then they finally have the collision in the center of the machine or some people described it as two smoke rings that collide. That’s one thing, the startup. And then the harder part, assuming you can master that is how do you keep it now operationally in the right condition throughout its life cycle when, as I said earlier, this stuff may want to get out of the engagement and becomes unwieldy. So both of those things have to get solved.
So we managed to do very well with the startup methodology. We built these systems that have two starters, they get primordial plasmas. They form that compound object and then we operate on it. The problem with that has been that variability isn’t very good. Fine tuning is sort of difficult and you also need a lot of extra equipment. You have these two end zones. where there’s a lot of complex, expensive gear from magnets to pulse power systems, which basically are systems that can take electric energy and store it up and then compress it into a really tiny, but very powerful, tiny meaning in time, very short, but very powerful pulse.
And that pulse creates these two primordial objects and fires at each other. All this equipment is complex. Needs maintenance, of course, needs space, cost. And then more importantly, when you run a power plant and you think about it, that starter thing that I just described, this collision process, you only need it once.
Assuming you run a power plant steady state, which is what we’re trying to do. You start it like once a year, let’s say. It’s like you have a car motor, and you run that motor once, and then the car runs for a year, but now you got to pay for the electric starter motor, the extra battery, all this kind of stuff, and you rarely, if ever use it.
So it feels already wrong from that perspective, but let’s argue that that system now stands also a bit in your way from a practical perspective, because let’s assume you get plasma to cook and actually reacting and now you have energy flowing out and this energy comes out in the form of particles and some radiation. And those particles, particularly that are streaming out with some energy from the fusion birth, they’re going to come out axially. And now they’re going to have to run through all these systems that sit there that you needed once to start the damn thing, right? And so that’s an impractical tool that creates engineering headaches.
McNiel: Can you explain axially so people understand what you’re talking about.
Binderbauer: Yeah, axially means it goes out towards the ends of this long cylinder, right? So think of this, you’ve got this cylindrical tube and then these particles are flowing out towards the ends of that tube, right? And so if at the ends of these tubes are these primordial starter systems and there’s stuff there that they can potentially interact with these outflowing particles and you want to minimize that.
And on the operation of a power plant that now runs 24/7, you’re for every second of the entire year that you’re running without a maintenance stop. You’re paying a price for the fact that for a few thousands of a second at its start, you needed all that stuff, but then you don’t need it anymore. And you can’t just magically disconnect that stuff. These are big systems. They’re part of the vacuum environment. So it’s not ideal. However that was the one thing that worked and we made that work better than anybody else that I know in the field. Reliable, predictable, controllable, adjustable. And that worked for us for a long time. I mean, the entire history of TAE was built on that.
But we always looked for something to be better. And, you know, we had this idea for a couple of decades, I mean, throughout the entire firm’s existence. And actually, as it turns out, we weren’t the first ones to think about that. There were people who dreamt about the idea. Is there a better way to start an FRC? Less violent, more controllable, more tunable, and none of this end gear that you need. So you can make the machine more compact axially, it is less expensive, and from an operational perspective, none of the headaches of streaming particles through these other sections and so on.
You want to collect all the energy that flows out. You want those converter systems to take that energy out effectively and efficiently as close to the core as you can. So you want to get rid of all this starter stuff and all these intermediate components.
And this is what we’re celebrating right now. We actually accomplished this, believe it or not, a quest of decades in the making, two decades roughly for TAE in its company’s history. And then prior to that, a couple of decades under DOE leadership and people around the world, glamoring to make an FRC more elegantly, more controllable, more practical. And that’s really hard to do. It’s a very hard problem. And it turns out once you solve it, the way we solved it, not only do you solve the starter problem. But you make the caging better and more controllable. And then the entire evolution from there, the steady state part now if you go to a power plant, you haven’t only solved what happens in those thousands of a second initially in that blink of an eye, but you’ve solved improvements. You’ve now brought yourself serious improvements for the entire rest of the lifetime of this system because you got rid of all this equipment, less maintenance on it, and most importantly upfront, a much lower cost of materials to build a functional reactor.
McNiel: So in summary, there’s a couple major things to celebrate here. Number one, you’ve mastered the FRC. You’re able to confine an FRC for an indeterminate amount of time, as long as you have energy to run it.
Number two, you simplified the vessel by removing a substantial amount of complicated hardware, costly hardware. So the machine is not as long as it once was. It’s not as complicated as it once was. It’s not as expensive as it once was. And you can form plasma in the central vessel and heat it up and energize it and keep it controlled.
Binderbauer: It’s also a lot more efficient. So more power will be sent out to the grid. And so the system will have a lower cost of electric production, not just because you have less equipment, but you’re also making more per moment, more power that becomes electrons to the grid.
McNiel: Well, Michl, thank you very much. This has been exceedingly informative and exciting.
Binderbauer: Thank you for keeping us in this really lively conversation. Much appreciated, Jim.
McNiel: Thanks for listening to Good Clean Energy. This season we’re going to unpack all the things that will make fusion a reality, the energy source that will power the planet for the next hundred thousand years. I’m pretty excited. I hope you are too. Thanks for listening. This is Jim McNiel.